Stem cells not only slow disease, they come with their own safety test
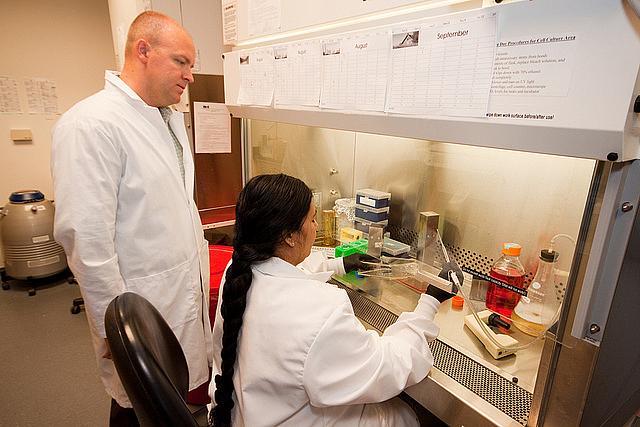
The speed of discovery in medical innovation exceeds that of any time in history, and “organoids” created from stem cells are one example. Called organoids because they are miniature human organs or partial versions of real organs, these tiny replicas are generated in a lab dish. Automobile crash-test dummies, when they slam into a wall at high speed, help researchers see how that would injure a live passenger; similarly, organoids can be used to safety-test stem cell treatments before they ever get near a human.
Organoids including mini-lungs and a mini-pancreas have been created. On the stem cell frontier, this is pivotal. Biologists and biochemists have historically had to settle for animal testing to gauge new findings. “Not previously accessible in humans,” is how one study author describes the momentousness of this unfolding discovery.
Organoids appear to be “self‐assembling” tissues; when their base is derived from stem cells and placed in a nutrient-rich lab dish, they cluster, organize and assemble themselves to actually resemble parts of real organs. They seem to know just where to go.
Organoids coaxed by scientists from various types of stem cells include mini-kidneys, mini-livers, mini-retinas and mini-intestines. Lung organoids can serve as a patient’s personalized cystic fibrosis drug‐testing tool. Indeed, “proxies” is the word the authors of Engineering Stem Cell Organoids use to describe them: “Organoid systems leverage the self-organizing properties of stem cells to create diverse, multi-cellular tissue proxies.”
Proving the safety of stem cell treatments is critical, and this is the value of these tiny versions of body parts: they are biochemical replicas of organs. Organoids are created by using a patient’s own stem cells as seeds. Exact twins of human tissue, when tested they will react to drugs, for example, the same way the donor patient’s living body will.
Organoids used as test models could decrease both animal testing and experimental surgeries on desperate patients. With organoids, no humans or animals are needed, and thus stem cells generate their own safety test. Moreover, the time and money needed to test organoids is drastically less than that needed for the traditionally years-long process of testing to meet U.S. Food and Drug Administration requirements.
Stem cells have been seen to reverse blindness, lung disease, intestinal maladies, heart problems and more. Their job is to repair damage following injury or disease. Stem cells can be found in many places — in bone marrow, skin, teeth and dental pulp. Some stem cells can regenerate, meaning they make copies of themselves, and they can become anything — a toe cell, or a backbone, or a nerve or a nose. The fact that stem cells can turn into any body part is key, and makes them extremely adaptable.
Organoids enable scientists to study both healthy and unhealthy cells. Those derived from healthy-tissue stem cells resemble the healthy tissue, while organoids derived from tumor cells resemble tumors. The FDA’s approval process could potentially be shortened if tumor organoids employed as disease models were used for testing.
A Dutch company, Hubrecht Organoid Technology, offers patients living biobanks. Using organoids created from cystic fibrosis patients, the company stores them in a biobank, and whenever there’s a new drug on the market to treat fibrosis, their organoids are tested to see if it is effective. Drugs work differently in every person, depending upon the particular biochemistry of the patient. The biobank concept has obvious benefit to patients; new drugs can be tested without patients even being involved. Their proxies do that for them.
The technique holds promise for conditions that deprive the lungs of oxygen. One stumbling block has been the inability to reproduce the scarring seen in lung disease like fibrosis. Using new techniques, stem cells are extracted from fibrosis patients’ lungs to make organoids. They grow into realistically dimensioned scars, tiny twins of those in the actual diseased lungs. With this fine-grained detail, treatment of scarring can be tailored to the specific patient whose scar cells spawned the organoid. “We can make thousands of reproducible pieces of tissue that resemble lung and contain patient-specific cells,” said one researcher.
“This near-physiological 3D model facilitates an accurate study” of a patient’s processes. Moreover, “an accurate study” can often be done in less time than conventional tests take. The promise of being able to test drugs on tumor organoids, and not humans or animals, is significant.
University of Toronto’s Dr. Molly Shoichet, in her 2016 article Why Scientific Breakthroughs Take Time to Move from Lab to Bedside, noted that “Studies often produce a lot of hope, but also frustration that the ‘product’ is not available to patients.” Her work to find a way to treat blindness using stem cells has been complicated, in need of exactitude and precision — and because it involves human eyeballs — loaded with ethical challenges that only time-consuming investigation can resolve. And the time it takes is nothing compared to the money it costs. Clinical trials that include volunteer patients for experimental surgeries are underway in the U.S., and stem cell trial treatments are taking place around the world.
The fact that organoids can self-assemble correctly in a relatively short time shows their “enormous self-organizing power,” according to one researcher. Organoids from colon cancer, pancreatic and prostate cancer patients’ stem cells have been developed and studied. The potential of organoids to cut short the “time to move from lab to bedside” is striking.
The time factor drives innovation. A Philadelphia company, BioBots, manufactures a type of 3-D printer that uses human tissue as a component to produce organoids. Instead of cartridges full of inks, BioBots’ printers contain human biomaterials, sometimes human stem cells. BioBots co-founder Danny Cabrera’s team develops the equipment that “prints” out heart tissue, liver tissues, skin tissues and others for research purposes. BioBots’ customers include scientists at New York’s Columbia University, University of Toronto, Massachusetts Institute of Technology, Rutgers University, University of Texas at Austin, Harvard and others.
BioBots printer users can inject into the printing process whatever biological material meets their needs. Operating layer by layer, the BioBot can output tiny 3-D versions of human tissue. Biologists then place this product into dishes, where over a period of time, with precise monitoring, the tissue transforms into an organoid with dimensions that mimic actual organs.
While studying biology and computer science at Penn State University, Cabrera noticed the prevalence of traditional, hidebound approaches, tools and lab equipment. “A lot of biology today is done by hand, with lots of manual work in the laboratory, sometimes using techniques and equipment not much different from what was developed 100 years ago. This leaves room for a lot of human error,” Cabrera said in a 2016 phone interview. He believed that a novel 3-D tool would be able to produce the same results biologists were producing one at a time, but be electronically programmed to produce more results, faster, and with the same quality every time, without human error. And he invented that tool.
The old, conventional methodology limited what one person could create. “A biologist will be working by hand, preparing materials in a dish, and producing a flat product, because they are limited by the flatness of the dish, their traditional methods, and the fact they are working by hand,” Cabrera says.
“We are focused on developing new tools for biologists,” he says of BioBots’ mission. “In the case of the 3-D printer, organoids can be produced, in larger numbers and with precision every time, that replicate the actual shape of the organ, so that an organoid liver, for example, will be the same shape and dimensionality as a real liver.” It might be tiny or microscopic in size, but it will not be cells lying flat in a dish; instead, it will resemble an actual liver with a round-edged triangular shape, and be biochemically the same as the human stem cell donor’s liver
Every innovation arrives astride pros and cons. One potential ethical issue is the type of tissue being generated. Growing organoids of human sex glands or reproductive organs in a lab dish will fuel concerns, notes Stanford University bioethical law professor Henry Greely. People are “not going to get upset about making a pancreas,” Greely said. “But the closer you come to making a human brain, the more issues get raised.”
Several British neuroscientists have developed brain organoids to study autism and schizophrenia. Their colleague Dr. Martin Coath, of Britain’s University of Plymouth, says they are creating a problem too big to overcome. Coath told the Daily Mail, “A human brain that was 'fully working' would be conscious, have hopes, dreams, feel pain, and would ask questions about what we were doing to it.”
Since organoids are often just portions of organs — or replicas of single, unattached organs — comprising enough tissue to test but not necessarily enough to function, science is a long way from producing a thinking mini-brain. Fears akin to Coath’s seem to have trended ever since Mary Shelley wrote “Frankenstein” in 1818. It is unlikely organoids are close to asking for updates, but their potential to slash drug-trial time, trial cost, and safety testing on desperately ill human volunteers or animals, is compelling.
[Photo by UC Davis via Flickr.]